In 2010, Alberto Hayek read a research paper that shocked him. Scientists at the J. Craig Venter Institute (JCVI) developed the first living organism controlled by a synthetic genome. The team had built artificial bacterial genomes, stuck them into bacterial cells of a different species and waited for those microbes to divide. The process bore viable, reproducing cells that solely relied on lab-created DNA. “My first thought was, ‘Why couldn’t they make a bacterium that makes insulin?’” recalls Hayek, now a professor emeritus and former pediatric endocrinologist at the University of California San Diego School of Medicine. Little did he know, an opportunity was about to land on his doorstep.
Health professionals have known for over 100 years that insulin treats the symptoms of diabetes and save lives. The hormone instructs the body to move glucose from the blood into cells when levels are too high. But with diminished insulin, blood sugar can skyrocket and lead to life-threatening complications. Everyone who has type 1 diabetes needs insulin because their bodies produce little to none; roughly one in four people with type 2 diabetes require injections too, as their insulin-producing pancreases wear out. Currently, about 8.4 million people in the United States use insulin, and that number will only rise as the rate of type 2 diabetes continues to climb.
The year after Hayek read the mind-boggling paper, a new construction site popped up near him. He noticed a familiar name on a billboard out front: J. Craig Venter Institute. The structure would become a state-of-the-art lab for the researchers, then equally split between California and Maryland, whose work he admired. Hayek cold-called the institute. “It was built three blocks from my house,” he says. “I thought it would be scientific malpractice not to approach them.”
In the fall of 2012, Hayek met John Glass, who co-authored the pivotal paper and leads the synthetic biology group at JCVI in San Diego. The duo brainstormed ways to merge their expertise in type 1 diabetes and synthetic biology over the following months. But as soon as they devised a potential plan with stem cells, Hayek consulted his lab neighbor, immunologist Richard Gallo, who proposed a different target: skin bacteria.
Now, the trio of scientists are engineering skin microbes into a diffuse network of continuous glucose monitors and insulin factories inside the body. The project comes amid recent slashes of sky-high prices for insulin, and both political and scientific efforts to scale up affordable options. People on Medicare started seeing insulin’s price capped at $35 per month last year thanks to the 2022 Inflation Reduction Act. And last March, California’s governor announced a drug manufacturer partnership to offer $30 vials of insulin.
But external insulin won’t be the only option for long. Restoring the body’s insulin production—through microbes or other means—is on the horizon. And the bacterial insulin pump project is a novel twist even in the emerging field of engineered bacterial therapeutics. “Everybody who’s doing this is carving their own path,” says Caroline Kurtz, Chief Development Officer of Synlogic, a Cambridge-based company using synthetic biology to develop therapeutics.
The out-of-the-box approach to insulin could provide a new option for people living with diabetes and add a fierce competitor to the largely monopolized insulin industry.
An Insulin Empire
Scientists first used insulin — extracted from cattle—as a type 1 diabetes treatment in 1922. Soon after, pharmaceutical companies began to sell cow and pig insulin. Animal insulin prevented complications from diabetes, but by the 1970s, experts worried there would soon be a shortage.
In 1978, biopharmaceutical company Genentech figured out how to make synthetic human insulin in the lab by inserting DNA into the bacterium Escherichia coli. Microbes began making insulin for us thanks to recombinant DNA technology. The young business partnered with pharmaceutical company Eli Lilly to scale up production, which began selling the synthetic insulin in the early 1980s.
When Glass was wrapping up his PhD about 10 years later, he believed scientists were about to crack the cure for type 1 diabetes. He was diagnosed with the disease in the fourth grade. And though his graduate work explored the genetics of viruses—far from the realm of metabolic disease—he stalked the field for any breakthroughs.
“There was a lot of really promising research going on,” says Glass. His colleagues suggested that he transition to type 1 diabetes research after graduate school, but he stuck with the principles of cellular life. “I didn’t want to go into anything that I thought was on the verge of being solved.”
But type 1 diabetes has not been solved as Glass had hoped. Today, pharmaceutical companies still use microbe-dependent technology to generate insulin. Three companies dominate the industry: Eli Lilly, Novo Nordisk, and Sanofi collectively control about 90% of the global insulin market.
With little competition, prices can soar. Until this year, many of the companies’ brand-name insulins went for almost $300 per 10-milliliter vial in the United States. Those price hikes have been largely driven by pharmacy benefit managers, companies that negotiate between pharmaceutical companies and insurers, according to a 2021 Senate Finance Committee report. The more money paid to these third parties by pharmaceutical companies to these third parties in rebates — calculated as a percentage of the list price — the better the company’s drug is placed on the list of medications insurers cover for patients. Four of the most popular insulins tripled in price from 2008 to 2017. An estimated 1.3 million insulin-using adults in the United States ration their doses and risk their health, according to a 2022 study.
“It should be a human right,” says Michael Weiss, a physician-scientist and biochemistry professor at the Indiana University School of Medicine in Indianapolis.
He notes that “insulin is cheap to mass produce in E. coli or yeast.” A 2018 analysis found that 10-milliliter vials of many insulin analogs—which each last a month, at most—should cost under $10 to make. Other companies could create generic versions using early research on human insulin analogs, as those patents have expired. But “generics would have to use the past-generation formulation chemistries, which are suboptimal from the perspective of 2023,” Weiss says.
Companies could also develop their own similar-acting versions of insulin analogs, called biosimilars. But establishing an appropriate biomanufacturing method can be costly, and it has been difficult for biosimilars to win approval from the U.S. Food and Drug Administration (FDA).
The three major pharmaceutical companies have now made insulin more affordable thanks to the 2021 American Rescue Plan Act. The drugmakers announced last March that they will cut the list prices of many insulin products by 65 to 80 percent and cap out-of-pocket expenses, effective this year.
Still, injecting insulin doesn’t cure the underlying damage. The pancreas’ “beta cells,” which produce insulin, get caught in the autoimmune crossfire of type 1 diabetes. The body attacks them, mistaking them as foreign invaders. One hot area of research involves replacing them with new beta cells, but the technique has some drawbacks.
What if we do something in between, giving individuals their own miniature insulin factories?
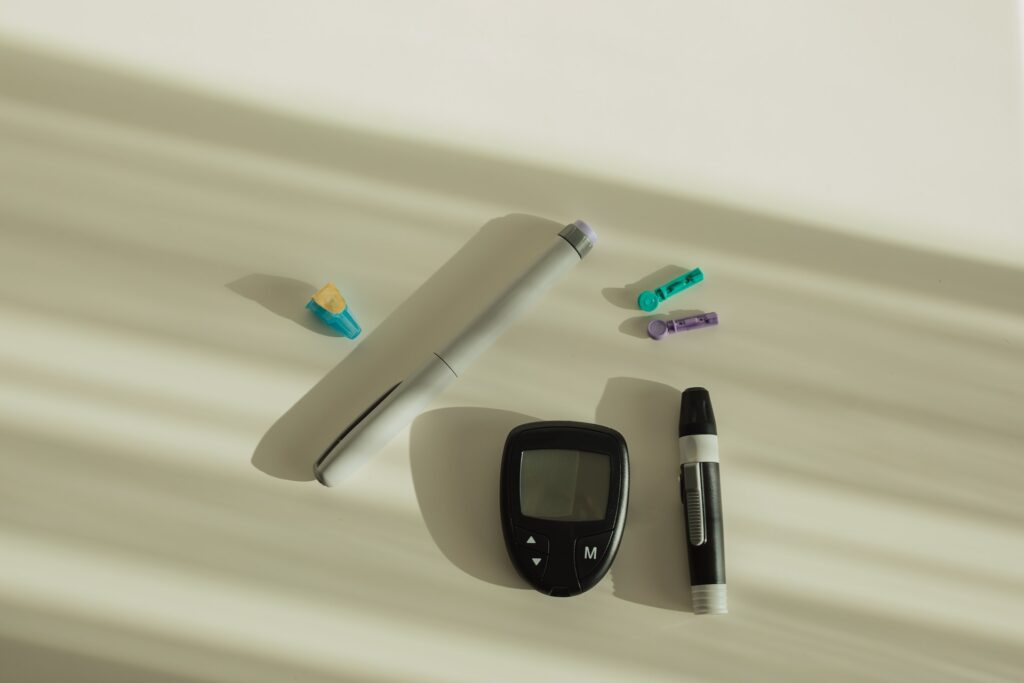
Microbe Monitors
Researchers first genetically engineered bacteria in the early 1970s, which led to their vital role in human insulin production. This technology progressed in parallel with interest in the microbiome—the vast world of microorganisms that live in and on us humans. The two fields finally merged in the mid-2010s, says Kurtz of Synlogic, who has written about engineered bacterial therapeutics. That “really spawned a lot of companies, like Synlogic and Ginkgo, to leverage this kind of technology to produce cells that could have therapeutic benefits.”
Today, a handful of engineered bacterial therapeutics are in clinical trials. Some genetically modified microbes instruct the immune system to attack diseased tissues, such as cancerous tumors, while others deliver a remedy, like giving a vital enzyme to patients who lack it.
“There’s a universe of things that you can do with this,” Kurtz says. “But it’s about really marrying those creative ideas with the practical aspects.”
Most engineered bacterial therapeutics act through the gut, since much of what we know about the human microbiome comes from bacteria that live there. But that route is a non-starter for diabetes. “Insulin wouldn’t survive well in the gut,” Glass says. Enzymes located there would degrade it.
The skin offers a new frontier thanks to a discovery made by Gallo. In 2013, his research group reported that friendly skin bacteria thrive deep within the organ’s layers, regions previously thought to be sterile. These bacteria reside so far below the surface—around two millimeters—that some can interact with blood vessels, says Gallo, a professor of dermatology at UC San Diego.
The bacterial insulin pump project focuses on altering Staphylococcus epidermidis’ DNA so that it senses a wearer’s raised blood sugar and churns out insulin accordingly. The bacterium is found in almost everyone, Gallo says. “Its genome has adapted to human skin, and human skin’s adapted to us,” he explains. “So we will permit a certain amount of Staph epidermidis to live on us.”
The skin offers a new frontier
That gives the potential treatment a leg up on work with cell replacement therapy. The company Vertex Pharmaceuticals, for instance, announced last June that two early-stage clinical trial participants with type 1 diabetes who received replacement beta cells—derived from stem cells—no longer need insulin injections. That same month, the FDA approved the first cell therapy for the disease, in which patients can receive pancreatic cells from deceased donors. But both options require individuals to suppress their immune systems to prevent their body’s defenses from attacking the foreign cells.
S. epidermidis would do the same job without the immune threat. And since the engineered bacterial insulin pumps just need access to the skin, they can be applied in a lotion.
Thus far, the team has synthesized a gene that instructs S. epidermidis to manufacture an insulin analog made of one amino acid chain. (Naturally-occurring human insulin contains two connected chains.) The single-chain insulin functions like the typical version but is more stable at warm temperatures and is easier for skin bacteria to construct, says Weiss, who developed the analog used in the work. “It’s just the perfect fit for the project.”
But functionality is just one piece of the puzzle. From the get-go, the team also needed a genetically encoded off-switch in case the microbe went haywire or was spread through skin-to-skin contact. Too much insulin leads to a drop in blood glucose, a condition just as dangerous as high blood sugar.
So the team removed the bacterium’s gene that encodes an ingredient for DNA called thymidine. This crucial component is only available in the lotion that also holds the bacteria. “This strain of Staphylococcus epidermidis has to be given this chemical because it can’t make it on its own,” Glass says. “That way it can’t escape.”
Still, to fully take over beta cells’ role, the bacteria must secrete insulin only in response to elevated blood sugar. The researchers are now searching for regions of DNA within S. epidermidis’ genome, called promoters, that can be used to build the engineered bacterium’s glucose sensor. In these promoter regions, proteins bind to trigger transcription of a neighboring gene. But finding them is an arduous task, says Kaisha Benjamin, a bioengineering PhD candidate at Stanford University who works on the project.
“It took me some time just trying to learn to work with the bug,” she says. The bacterial strain she works with has a thick, rigid cell wall that’s tough to crack, making it difficult to extract nucleic acid.
She has since found a workaround and identified some genes that have increased messenger RNA levels in response to a glucose bath. Now, Benjamin is hunting for the promoters that increase glucose-responsive expression. Placing the best one or two promoters into S. epidermidis’ genome upstream of the added insulin gene should make the bacterium pump out an appropriate amount of insulin only when blood sugar is too high.
Treating diabetes is “a balancing act,” Glass says. “You have to be just at that sweet spot — the exact right amount of insulin so that you don’t get hypoglycemia or hyperglycemia.”
Long Road Ahead
The project has made key strides over the last decade, but many challenges remain. Besides the in-progress glucose monitoring portion, the engineered bacteria currently pump out less insulin than expected. “We’re definitely not making enough,” says Yo Suzuki, an associate professor and synthetic biologist at JCVI. The researchers are now tweaking the genetic machinery to boost insulin output.
The researchers also remain unsure if one engineered bacterial strain will work for all insulin users, or if they’ll need personalized versions. “Different people probably all harbor multiple different strains of S. epidermidis,” Glass explains.
But even if they accomplish all the required technical feats, making the treatment available to patients will take time, effort and “a staggering amount of money,” for development and regulatory approval, Glass says. That raises eventual prices, though more competition could help keep costs reasonable.
Out of the products widely available today, automatic insulin pumps most closely resemble the body’s normal release. But it requires a pricey pump and continuous blood glucose monitor, both of which require constant maintenance and insulin refills. Moving insulin manufacturing from bioreactor facilities to the skin of individual users could make it cheaper and more effective than this current best option, Glass says—and “the biological control that the bacteria offer should be better.”
It’s a unique marriage between a traditional system and new technology. “I don’t know whether it’ll work in the end,” Weiss says. “But it’s one of these bold, disruptive ideas that makes it exciting to be in science.”